Collaboration the Key to Unlocking Cells’ Secrets
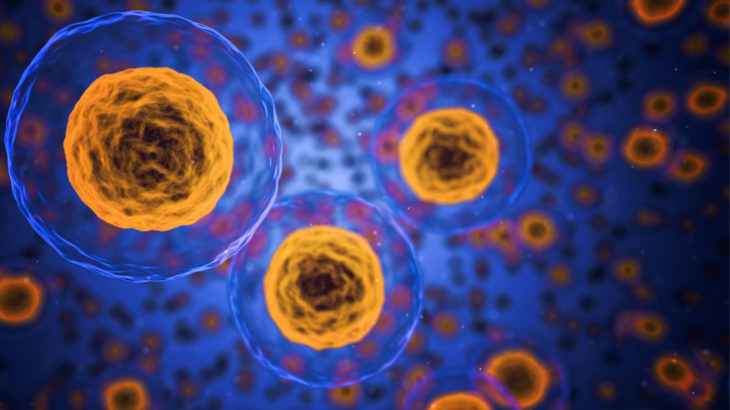
With the NHS celebrating its 70th birthday this year, it’s an appropriate time to reflect on the vital role of drug discovery in the never-ending drive to improve healthcare – and on the ground-breaking research that makes such discoveries possible.
Research outcomes can be strengthened hugely by working across disciplines and by combining complementary skills across institutions. An excellent example of both is a well-established collaboration between teams at Oxford University and UCL that’s generating deeper understanding of the innermost workings of the central nervous system.
Harnessing a combination of cutting-edge experimental and computational techniques, the research is delivering insights with the potential to support development of new breeds of drugs targeting inflammatory pain, Parkinson’s Disease and other serious conditions.
A Journey of Discovery
When a new drug appears on pharmacy shelves, it frequently marks the end of a long and extraordinary journey. In some cases, the story can be traced back many years to fundamental research that helped lift the lid on the mysterious molecular-level processes that make the body function (or malfunction). Without this ever-extending platform of core knowledge, the development of many effective new therapies simply wouldn’t be possible.
“We’re using leading-edge experimental techniques to dig deeper and deliver new insights into receptor proteins”
Professor Lucia Sivilotti, UCL
Take ‘receptor’ proteins. These perform a vital role in cells, binding with specific molecules outside the cell when they come into contact with them and, as a result, altering how the cell functions. For example, the receptor protein may change shape, opening a channel into the cell that enables electrically charged ions to flow in and out – a process fundamental to the transmission of nerve impulses in the brain and the central nervous system.
Many drugs already target these proteins. However, many of these were discovered by screening large numbers of chemicals to find ones that were effective, rather than by designing chemicals to target a known receptor structure. Huge potential remains untapped, then, not least with regard to modifying the behaviour of specific receptors to help reduce pain or treat neurological conditions. But this requires a more detailed grasp of how they work.
“Here at UCL, we’re using leading-edge experimental techniques to dig deeper and deliver new insights into receptor proteins,” says Professor Lucia Sivilotti. “We see function at single-molecule level. But even these techniques have limits in terms of attributing changes in the receptor’s activity to changes in the receptor’s atomic-scale structure.”
That’s why her group has developed a productive relationship with Professor Phil Biggin’s computational biochemistry group at Oxford. “The computer modelling and processing power now available means we can simulate with unprecedented accuracy the molecular structure of receptor proteins and the way they bind and change,” Professor Biggin says. “Simulations can also shed light on experimental data that otherwise can’t be explained.”
Through the Pain Barrier
With Medical Research Council funding, this fruitful collaboration has, for example, unpicked some previously impenetrable secrets surrounding the human glycine receptor. This protein performs important functions in a number of physiological processes, most especially in helping to reduce neuron-firing in the brain stem and spinal cord. (Neurons are the basic constituents of the central nervous system.)The Oxford/UCL team set out to reveal how glycine (an amino acid present in high concentrations in skin and muscle tissue) binds to this receptor – knowledge that could underpin pharma companies’ development of more effective painkillers, for example.
Lucia Sivilotti takes up the story: “We needed a framework to help us build on and interpret the experimental data we were generating. A key problem was that few structural data are available for glycine receptors, and none for the human glycine receptor. This prevented us examining the molecule’s detailed structure in the lab and presented a major barrier to working out precisely how the protein behaves and how it affects the process of neuron transmission.”
“These are just the sort of detailed discoveries that can provide an excellent starting point for the rational design of innovative drug therapies”
Professor Phil Biggin, University of Oxford
The Oxford group provided the solution. “We built a model of the receptor’s structure that was consistent with – and was validated using – the experimental data obtained by our colleagues at UCL,” Phil Biggin explains. “We then used the model to predict the molecule’s behaviour and explore some intriguing phenomena revealed by the lab experiments.”
This close interaction of computational and experimental science paid impressive dividends. Not only was the model successfully developed, tested and deployed; the team’s work also led to important discoveries such as the presence of:
- A water molecule in the zone where the binding between the glycine molecule and the human glycine receptor takes place.
- A residue of the amino acid serine 129, which was found to play a central role in the binding process.
“These are just the sort of detailed discoveries that can provide an excellent starting point for the rational design of innovative drug therapies,” Phil Biggin confirms.
New Tools, New Rules
Such breakthroughs also underline the value of the game-changing computing capabilities increasingly harnessed by UK researchers. Phil Biggin’s group have been able to maximise the impact of their modelling and simulation skills by harnessing powerful high-performance computing (HPC) hardware available via Oxford’s Advanced Research Computing (ARC) resource and the Edinburgh-based ARCHER national HPC facility.
Looking ahead, the group will increasingly utilise JADE (Joint Academic Data Science Endeavour), a cutting-edge new facility developed with EPSRC support by an Oxford-led consortium that also includes KCL, QMUL, Southampton and UCL, plus non-SES members. In fact, one of the Biggin’s group was an early tester of JADE and has helped to write the guidelines on running simulations using the facility.
“Ever-improving computing capabilities open up phenomenal possibilities,” Phil Biggin concludes. “In particular, the molecular-level information that models can now generate will aid development of drugs that work in much more subtle ways.
“Traditionally, drugs targeting neurological conditions have generally been something of a blunt instrument, designed to completely block a specific physiological process, for example. The detailed knowledge we can now produce will make it easier to identify ways of simply tweaking how molecules behave in order to repair the slight imbalances that often lie at the root of neurological conditions.
“It’s no exaggeration to say that the computational tools we can deploy today have the potential to help rewrite the rulebook on drug discovery.”
Further Information
- Biggin group homepage: http://sbcb.bioch.ox.ac.uk/bigginlab/
- Sivilotti group homepage: http://www.onemol.org.uk/
- Phil Biggin on Twitter: https://twitter.com/philbiggin
- UCL Department of Neuroscience, Physiology and Pharmacology: https://twitter.com/uclnpp
Agonist and Antagonist Binding in Human Glycine Receptors
Professor Lucia Sivilotti
Professor Phil Biggin